The Independent's journalism is supported by our readers. When you purchase through links on our site, we may earn commission.
New insight into elusive antimatter can help unravel universe’s mysteries
New research has compared hydrogen and antihydrogen up to ten decimal places for the very first time
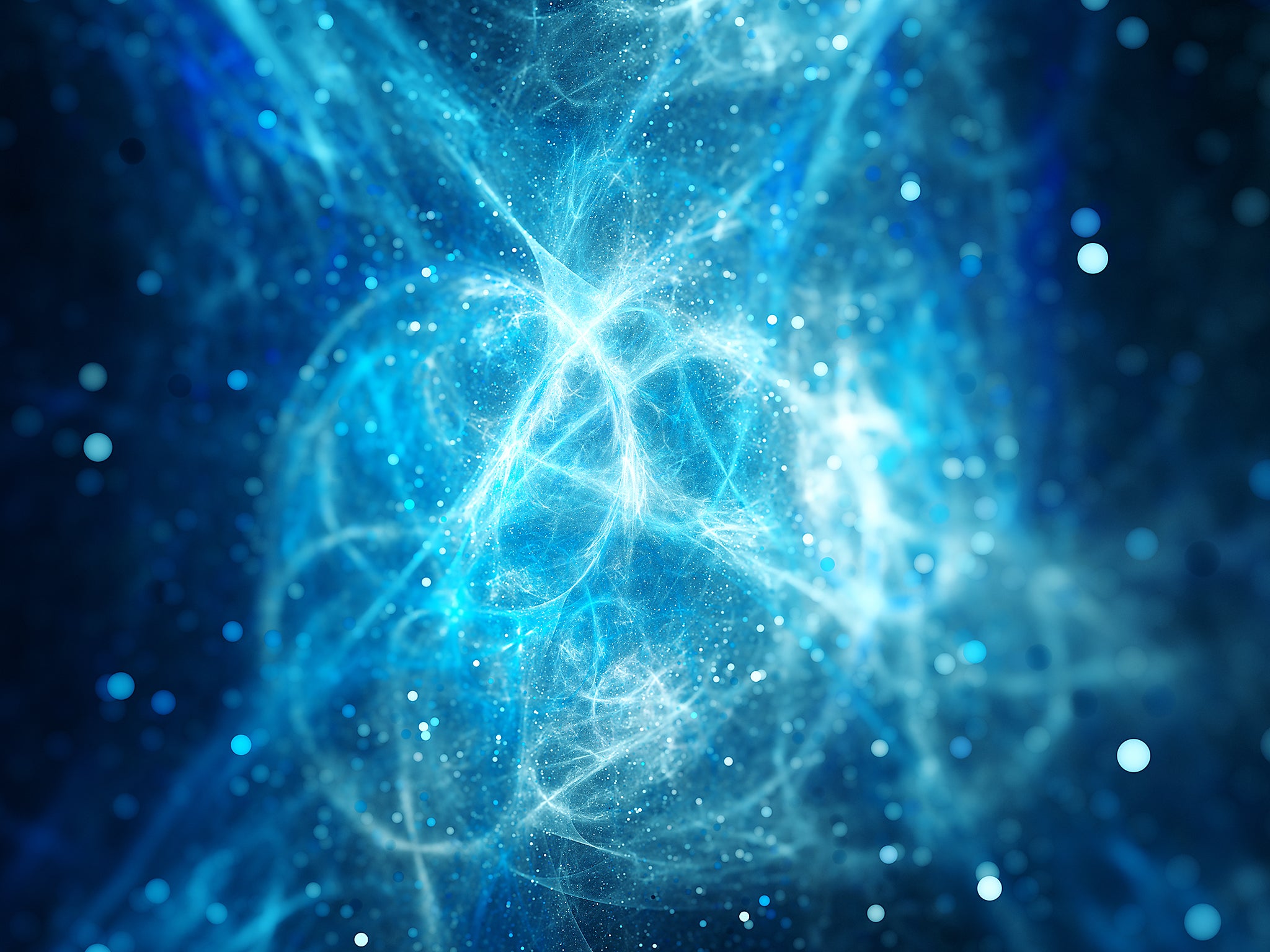
Your support helps us to tell the story
From reproductive rights to climate change to Big Tech, The Independent is on the ground when the story is developing. Whether it's investigating the financials of Elon Musk's pro-Trump PAC or producing our latest documentary, 'The A Word', which shines a light on the American women fighting for reproductive rights, we know how important it is to parse out the facts from the messaging.
At such a critical moment in US history, we need reporters on the ground. Your donation allows us to keep sending journalists to speak to both sides of the story.
The Independent is trusted by Americans across the entire political spectrum. And unlike many other quality news outlets, we choose not to lock Americans out of our reporting and analysis with paywalls. We believe quality journalism should be available to everyone, paid for by those who can afford it.
Your support makes all the difference.The universe is made of both matter and antimatter – this is what physicists since the 1930s have believed. While we are well aware of what the physical matter is, antimatter has remained an elusive substance.
But that is about to change: I have been involved with a group of scientists whose newly published research on antihydrogen – the antimatter counterpart of hydrogen – heralds a new era in the effort to understand more about antimatter and how it has managed to evade us.
So what is antimatter? In the late 1920s, theoretical physicist Paul Dirac predicted the existence of “mirror” particles – opposite counterparts to the already known electrons and protons. These mirror particles had opposite charge so they were a positive electron and a negative proton – later named positron and antiproton. The positron was discovered a few years later in 1932, but it took scientists until 1955 to discover the antiproton.
The discovery was tricky as antimatter doesn’t seem to be prevalent in the universe. In fact, the antiproton was only discovered because a particle accelerator was built specifically to create them.
According to Einstein’s famous equation E=mc2, mass can be converted to energy and vice versa. The accelerator worked by supplying enough energy to create antiprotons by converting energy to mass. Mass is a compact holder of energy, but not all of it can normally be released – even a nuclear weapon only releases a tiny fraction of the energy of its mass.
When a particle and its antiparticle are brought together, they annihilate each other – that is, they collide and disappear – and all their mass energy is released in a burst of light. The opposite is also true: with sufficient energy, we can create matter, but like annihilation, this process is also symmetric, so matter and antimatter will always be created in equal quantities.
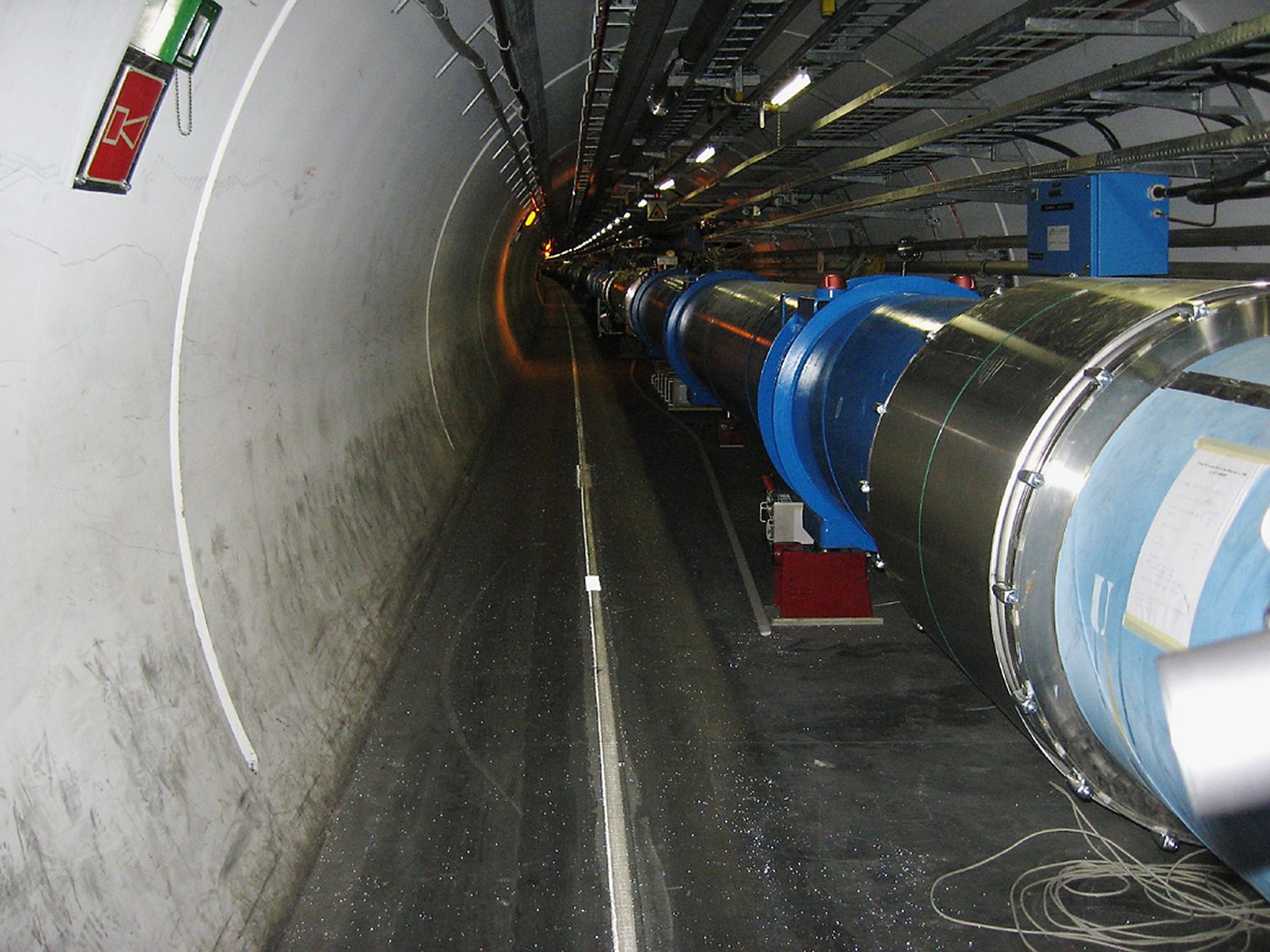
This is the process by which the first antiproton was created, and it is still what we use today. But it is incredibly inefficient: in a typical creation process at CERN’s antiproton decelerator, about a million protons are collided with a metal target to yield a single antiproton.
Why is it important?
Physicists believe that the universe was created in the Big Bang billions of years ago, and in particular that it started out so hot and tiny that no particles could form at the very start. As this primordial energy soup cooled, particles and antiparticles formed in equal quantities. But less than a second after the Big Bang, something happened that caused an asymmetry, leaving a small excess of matter behind. So where did all the antimatter go? We simply don’t know – this is one of the greatest mysteries of physics.
There is no explanation for this asymmetry, in fact we cannot explain how we can be here, as this asymmetry is required for the universe we know to exist.
Despite many lifetimes of careful observation of the skies, so far no clues have been found to tell us why there is this asymmetry between matter and antimatter. Many scientists have looked in various ways at antimatter, to try to unravel if there is some fundamental difference between it and matter that could have caused this asymmetry. The traditional method is to look at the results of high-energy collisions, for example by using the Large Hadron Collider at CERN. However, we are now pursuing a very promising alternative to this.
Hydrogen is the most abundant substance in the universe and consists of just one electron and one proton. It’s fair to say that it is the best understood system in physics, both experimentally and theoretically. It also played a key role in the discoveries that led to quantum mechanics. The internal properties of hydrogen have been studied to staggering precision using lasers, and the energy difference between its ground state and the first excited state – where it has excess energy – is known in detail. It is similar to a guitar string – its ground state means the string is not vibrating and an excited state means it is. The more it is vibrating, the more excited it is.
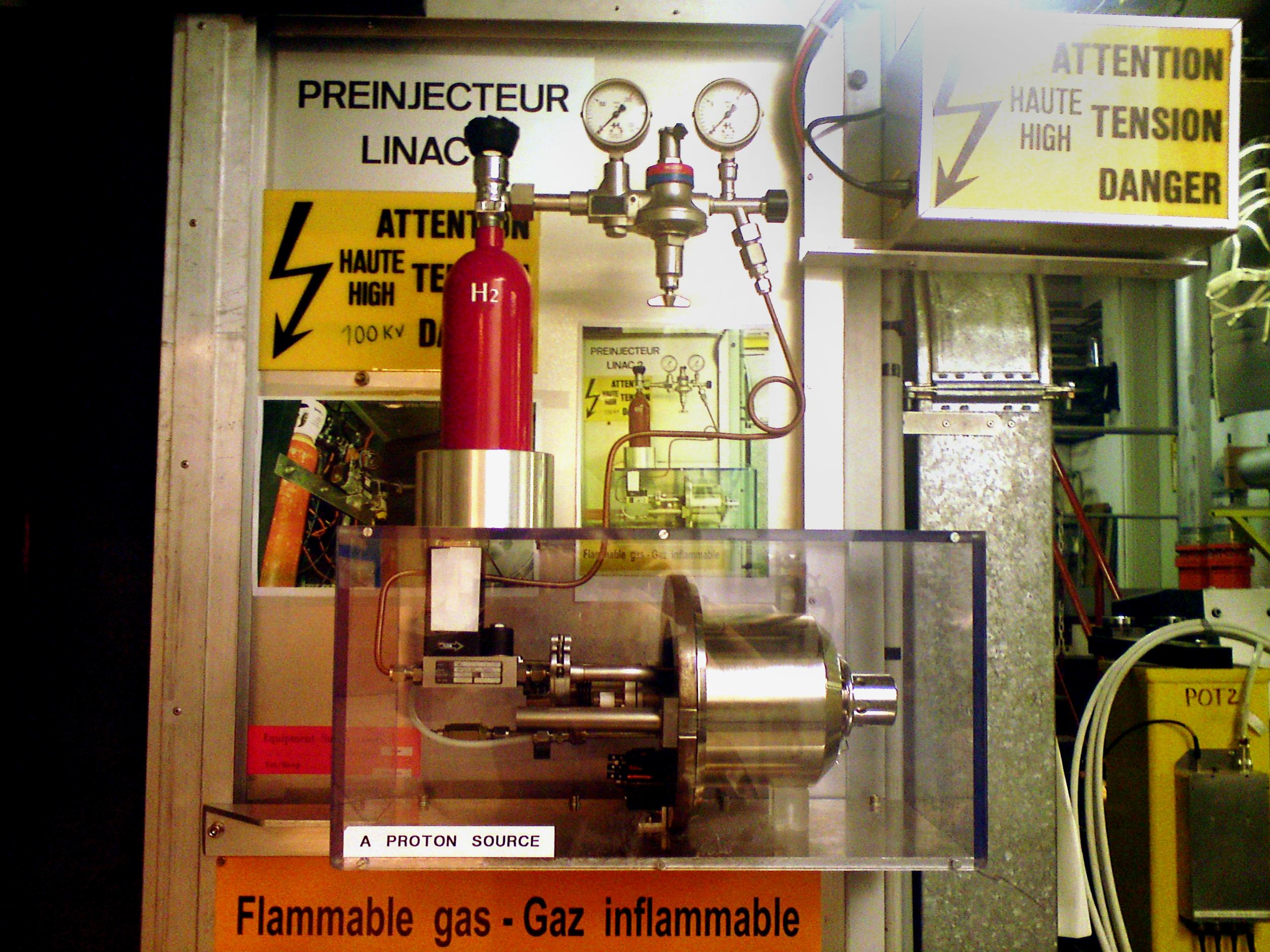
For more than 30 years, researchers have been working to unravel the mystery of antimatter using antihydrogen, and we have just accomplished a major breakthrough.
What we have just done is to shine laser light on trapped antihydrogen atoms and excite them to their first excited state. We can study their behaviour as they gain energy from the laser light – or get excited. Eventually, they break apart – that’s how we could tell they had absorbed the energy.
One reason it has been so hard to do this is that antimatter is always annihilated when it encounters matter. This makes it challenging to store – you can’t just put it in a bottle. However, we have already managed to make and hold antihydrogen using an array of electromagnets that can constrain it, which allowed us to do this research.
This very first measurement allows us to compare hydrogen and antihydrogen with unprecedented precision – indeed, it is the most precise comparison of an atom and an antiatom ever made.
Using this measurement, they look identical, and though that was to be expected, it is the first experimental confirmation. For now, the mystery of the elusive antimatter continues – but it is something we are continuing to pursue.
Niels Madsen is a professor of physics, at Swansea University. This article first appeared on The Conversation (theconversation.com)
Subscribe to Independent Premium to bookmark this article
Want to bookmark your favourite articles and stories to read or reference later? Start your Independent Premium subscription today.
Join our commenting forum
Join thought-provoking conversations, follow other Independent readers and see their replies
Comments